Quantum Computing: is a cutting-edge field of computing that leverages the principles of quantum mechanics to process and manipulate information in fundamentally different ways than classical computers. Classical computers, which include the devices we use daily like smartphones and laptops, use bits as the basic unit of information, where each bit represents either a 0 or a 1. In contrast, quantum computers use quantum bits or qubits.
Types of Quantum Computing
Quantum computing is an evolving field with several different approaches and types of quantum computers being developed.
Here are some of the main types of quantum computing:
- Quantum Gate-Based Quantum Computing: This is the most well-known and widely studied type of quantum computing. Gate-based quantum computers use qubits and quantum gates to perform calculations. They manipulate qubits through operations similar to classical logic gates (AND, OR, NOT) but with quantum properties like superposition and entanglement. Prominent quantum computing companies, such as IBM, Google, and Rigetti, are developing gate-based quantum computers.
- Quantum Annealing: Quantum annealing is an approach to quantum computing that focuses on solving optimization problems. Quantum annealers use qubits to explore the energy landscape of a problem and find the lowest-energy configuration, which corresponds to the optimal solution. Companies like D-Wave Systems have developed quantum annealers for this purpose.
- Topological Quantum Computing: This is a theoretical approach to quantum computing that relies on exotic particles called non-abelian anyons, which are predicted to obey nontrivial braiding statistics. These anyons could be used for fault-tolerant quantum computation, but practical implementations are still in the early stages of research.
- Quantum Simulation: Quantum simulators are specialized quantum computers designed to simulate the behavior of complex quantum systems, such as molecules and materials. They are valuable for tasks like drug discovery and materials science.
- Quantum Communication: While not strictly quantum computing, quantum communication involves using quantum properties like entanglement for secure communication. Quantum key distribution (QKD) is a notable application of quantum communication for secure encryption.
- Quantum Cryptography: Quantum computers have the potential to break many classical encryption methods. Quantum cryptography is a field focused on developing quantum-resistant cryptographic techniques to secure communications in a post-quantum world.
- Hybrid Quantum-Classical Computing: Many practical quantum computing applications may involve a combination of classical and quantum computing. Hybrid quantum-classical systems leverage the strengths of both classical and quantum processors to solve complex problems.
- Quantum Machine Learning: Quantum computing has the potential to enhance machine learning algorithms and solve certain problems more efficiently. Quantum machine learning explores how quantum computers can accelerate tasks like optimization, data analysis, and pattern recognition.
- Quantum Cloud Computing: Some companies are working on making quantum computing resources accessible via the cloud. Users can access and run quantum algorithms on remote quantum computers over the internet.
These are some of the primary types of quantum computing, and the field is still evolving with ongoing research and development. The choice of quantum computing approach depends on the specific problem being addressed, the available hardware and technology, and the desired outcome.
Features of Quantum Computing
Quantum computing is a revolutionary paradigm of computation that offers several distinctive features and advantages over classical computing.
Here are some of the key features of quantum computing:
- Superposition: Quantum bits or qubits can exist in multiple states simultaneously. Unlike classical bits, which can be either 0 or 1, qubits can represent both 0 and 1 or any combination of states in between. This enables quantum computers to perform parallel computations on a vast scale, potentially solving certain problems much faster than classical computers.
- Entanglement: Quantum entanglement is a phenomenon where the quantum states of two or more qubits become correlated in such a way that the state of one qubit is dependent on the state of another, even when separated by large distances. This property allows for the creation of highly interconnected and interdependent qubit systems, enabling more powerful computations and communication protocols.
- Quantum Interference: Quantum interference is a fundamental quantum phenomenon that allows quantum computers to exploit the wave-like nature of quantum particles. Quantum algorithms can use interference to amplify the probability of correct answers while suppressing incorrect ones, leading to enhanced computational efficiency.
- Exponential Speedup: Quantum computers have the potential to solve certain problems exponentially faster than the best-known classical algorithms. This advantage is particularly evident in applications like factoring large numbers, optimizing complex systems, and simulating quantum systems.
- Quantum Tunneling: Quantum tunneling is a property that allows quantum computers to explore energy landscapes more effectively, which is valuable for solving optimization problems. Quantum annealers, for instance, take advantage of this property to find optimal solutions efficiently.
- Security: Quantum computing introduces both opportunities and challenges for security. While quantum computers can break many classical encryption methods, they also enable the development of quantum-resistant cryptographic techniques like quantum key distribution (QKD) for secure communications.
- Quantum Error Correction: Quantum computers are susceptible to errors due to decoherence and noise. Quantum error correction codes and techniques are being developed to protect quantum information and enable fault-tolerant quantum computation.
- Hybrid Computing: Quantum computers can be integrated with classical computers in a hybrid computing model. This allows classical processors to handle tasks they are well-suited for while offloading certain subproblems to quantum processors, maximizing the advantages of both technologies.
- Quantum Machine Learning: Quantum computing has the potential to accelerate machine learning algorithms and improve data analysis, pattern recognition, and optimization tasks. Quantum machine learning is an emerging field that explores these possibilities.
- Quantum Cloud Computing: Some companies are working on making quantum computing resources accessible via the cloud, allowing researchers and organizations to access quantum computers remotely for experimentation and computation.
These features of quantum computing highlight its potential to revolutionize various fields, from cryptography and materials science to optimization and machine learning. However, it’s important to note that quantum computing is still in its early stages of development, and practical, large-scale quantum computers are not yet widely available. Researchers and organizations are actively working on overcoming the technical challenges to harness the full power of quantum computing.
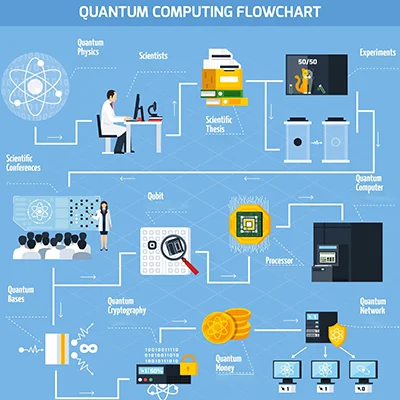
Benefits of Quantum Computing
Quantum computing holds the promise of revolutionizing various fields and industries by offering unique advantages over classical computing. While practical, large-scale quantum computers are still in the early stages of development, their potential benefits are significant.
Here are some of the key benefits of quantum computing:
- Exponential Speedup: Quantum computers have the potential to solve certain problems exponentially faster than classical computers. This can lead to significant breakthroughs in areas such as cryptography, optimization, drug discovery, materials science, and more. For example, factoring large numbers, which is crucial for breaking many encryption schemes, could become much faster with quantum computers.
- Quantum Simulations: Quantum computers can simulate quantum systems much more efficiently than classical computers. This capability is vital for understanding and designing molecules, chemical reactions, and materials at the quantum level. It can lead to the development of new drugs, materials, and energy solutions.
- Enhanced Machine Learning: Quantum computing can accelerate machine learning algorithms, enabling faster data analysis, pattern recognition, and optimization. This can have applications in fields like finance, healthcare, and artificial intelligence.
- Quantum Cryptography: Quantum computing presents both challenges and opportunities for cryptography. While it can break many classical encryption methods, it also enables the development of quantum-resistant cryptographic techniques like quantum key distribution (QKD), which offers unprecedented security for communication.
- Advanced Optimization: Quantum computers excel at solving optimization problems, which are prevalent in logistics, supply chain management, financial modeling, and more. Quantum optimization algorithms can lead to more efficient resource allocation and cost savings.
- Material Discovery: Quantum computers can assist in the discovery of novel materials with unique properties, potentially revolutionizing industries such as electronics, energy storage, and manufacturing.
- Pharmaceutical Research: Quantum computing can expedite the drug discovery process by accurately simulating molecular interactions and predicting drug effectiveness. This can lead to the development of more effective drugs and treatments.
- Climate Modeling: Quantum computers can perform complex climate simulations and help researchers better understand climate change, leading to more accurate predictions and informed climate policies.
- Supply Chain Optimization: Quantum computing can optimize complex supply chains, reducing waste, transportation costs, and environmental impact.
- Financial Modeling: Quantum computing can improve risk assessment, portfolio optimization, and fraud detection in the financial sector.
- Space Exploration: Quantum computing can help solve complex problems related to space exploration, such as trajectory optimization, satellite communication, and data analysis from space missions.
- Healthcare and Genomics: Quantum computing can analyze vast genomic datasets more efficiently, leading to personalized medicine, disease prediction, and drug development breakthroughs.
It’s important to note that quantum computing is still in its infancy, and practical, scalable quantum computers are not yet widely available. Overcoming technical challenges related to error correction, stability, and scalability is an ongoing area of research. However, as quantum computing technology advances, its potential to bring about significant benefits in various domains becomes increasingly apparent.
How Does it work!
Quantum computing works fundamentally differently from classical computing, harnessing the principles of quantum mechanics to process information. Instead of using classical bits (which can be either 0 or 1), quantum computers use quantum bits or qubits.
Here’s a simplified overview of how quantum computing works:
- Quantum Superposition: One of the key principles of quantum computing is superposition. While classical bits can only be in one of two states (0 or 1), qubits can exist in multiple states simultaneously. This means a qubit can represent 0, 1, or any combination of 0 and 1 at the same time. Superposition allows quantum computers to explore many possibilities in parallel.
- Quantum Entanglement: Another critical principle is entanglement. When qubits are entangled, the state of one qubit becomes dependent on the state of another, even when they are physically separated. This interconnectedness enables the creation of highly correlated qubit systems.
- Quantum Gates: Quantum computers use quantum gates to manipulate qubits and perform calculations. These gates are similar in concept to classical logic gates (AND, OR, NOT), but they operate on quantum states, taking advantage of superposition and entanglement. Quantum gates can create complex operations that classical gates cannot.
- Quantum Measurement: When a quantum computer performs a measurement on a qubit, it collapses the superposition state into a definite 0 or 1. The probability of measuring each outcome depends on the quantum state’s coefficients in superposition.
- Quantum Interference: Quantum interference is a crucial aspect of quantum algorithms. It leverages the wave-like properties of quantum states to enhance the probability of obtaining the correct answer while reducing the probability of incorrect answers. This interference amplifies the efficiency of quantum algorithms.
- Quantum Algorithms: Quantum computers use specialized quantum algorithms to solve specific problems more efficiently than classical algorithms. These algorithms take advantage of quantum properties like superposition, entanglement, and interference to perform calculations faster than classical counterparts.
- Quantum Error Correction: Quantum computers are susceptible to errors due to factors like decoherence and noise. Quantum error correction codes and techniques are developed to protect quantum information and enable fault-tolerant quantum computation.
- Quantum Gates Sequences: Quantum computations involve sequences of quantum gates that manipulate qubits. The order and combination of gates are designed to perform specific tasks, such as factorization, optimization, or simulation.
- Hybrid Quantum-Classical Computing: Practical quantum computers are currently limited by factors like qubit stability and error rates. To address these limitations, researchers often use a hybrid approach, combining classical and quantum processors. Classical computers handle tasks they are well-suited for, while quantum processors accelerate specific subproblems.
- Quantum Algorithms: Quantum algorithms, such as Shor’s algorithm (for factoring large numbers) and Grover’s algorithm (for unstructured search), demonstrate the potential of quantum computing by solving problems exponentially faster than classical counterparts.
It’s important to note that quantum computing is a complex and rapidly evolving field. Building practical quantum computers and designing quantum algorithms requires overcoming significant technical challenges, such as qubit stability, error correction, and scalability. Researchers and organizations worldwide are actively working on advancing quantum computing technology to unlock its full potential.
Can quantum computers solve all problems?
Quantum computers are powerful and have the potential to solve certain problems exponentially faster than classical computers. However, they are not a panacea and cannot solve all problems.
Here are some key points to consider:
- Quantum Advantage: Quantum computers excel at solving specific types of problems, including integer factorization (e.g., breaking RSA encryption), database search (e.g., Grover’s algorithm), and simulating quantum systems (e.g., quantum chemistry simulations). For these problems, quantum algorithms can provide a significant advantage in terms of computational speed.
- Exponential Speedup: The advantage of quantum computers is often described as an “exponential speedup.” This means that as the size of the problem grows, the advantage of quantum computing over classical computing becomes exponentially greater. In contrast, for many other problems, the advantage may be negligible or even non-existent.
- Quantum Algorithms: The power of quantum computing is closely tied to the development of quantum algorithms that exploit quantum principles like superposition and entanglement. Not all problems have efficient quantum algorithms, and researchers are still exploring which problems can benefit from quantum computation.
- Physical Limitations: Building practical, large-scale quantum computers is a complex and ongoing challenge. These machines are susceptible to errors caused by factors like decoherence and noise. As a result, they may not be well-suited for all types of computations, especially those requiring high levels of precision and error tolerance.
- Classical Algorithms: Classical computers have proven to be highly effective for many types of problems, including numerical simulations, data analysis, graphics rendering, and more. Quantum computers are not expected to outperform classical computers in all of these domains.
- Hybrid Computing: In practice, researchers often use a hybrid approach that combines classical and quantum computing. Classical computers handle tasks they are good at, while quantum processors accelerate specific subproblems. This approach is expected to be common as quantum technology matures.
- Security Implications: While quantum computers have the potential to break many classical encryption methods (such as RSA and ECC), they can also enable the development of quantum-resistant cryptographic techniques. The field of post-quantum cryptography is actively addressing this challenge.
In summary, quantum computers are a powerful tool with the potential to revolutionize computing for certain problems. However, they are not a universal solution and are unlikely to replace classical computers entirely. The choice of whether to use a quantum or classical computer depends on the specific problem to be solved, the available quantum hardware, and the efficiency of quantum algorithms for that problem. Researchers continue to explore the boundaries of quantum computing and its practical applications.
What is the future of quantum computing in India?
The future of quantum computing in India holds significant potential, but it depends on various factors, including investments in research and development, collaboration with international partners, and the adoption of quantum technologies in various sectors.
Here are some key aspects of the future of quantum computing in India:
- Research and Development: India has a growing presence in quantum research, with several academic institutions and research centers actively engaged in quantum physics, quantum information science, and quantum computing. Continued investments in R&D are crucial for advancing quantum technologies.
- International Collaboration: Collaboration with leading global institutions and organizations in the field of quantum computing is essential. India can benefit from partnerships with established quantum research centers and companies to access cutting-edge knowledge and technologies.
- Skilled Workforce: Building a skilled workforce in quantum computing and quantum information science is vital. This involves training researchers, engineers, and developers in quantum programming and quantum algorithms.
- Government Initiatives: Government support is crucial for the growth of quantum computing in India. Initiatives such as funding for quantum research, establishing quantum computing centers, and formulating quantum policies can help foster the development of the quantum ecosystem.
- Quantum Startups: The growth of quantum startups in India can drive innovation and commercialization of quantum technologies. Encouraging entrepreneurship and providing support for quantum-related startups is essential.
- Education and Awareness: Promoting awareness and education about quantum computing and its potential applications is vital. This can include educational programs, workshops, and outreach activities to engage students, researchers, and the general public.
- Applications in Key Sectors: India can explore the use of quantum computing in sectors such as healthcare (drug discovery, genomics), finance (portfolio optimization, risk assessment), logistics (supply chain optimization), and defense (cryptography, simulations).
- Quantum-safe Cybersecurity: As quantum computers advance, India should focus on developing and adopting quantum-resistant cryptographic techniques to secure its critical infrastructure and communications.
- Quantum Policy Framework: Developing a comprehensive policy framework for quantum computing and technology is essential for addressing regulatory, security, and ethical aspects of quantum technologies.
- Global Competitiveness: India has the opportunity to become a global player in quantum computing and technology. Strategic investments and collaborations can help India position itself as a leader in this emerging field.
The future of quantum computing in India depends on its ability to leverage its research strengths, foster innovation, and create an ecosystem that supports quantum technology development and adoption. As quantum computing continues to advance, it has the potential to address complex challenges and drive innovation across various industries in India and on the global stage.
Internet of Things: (IoT) Benefits & Building The Urban Future
Cloud Computing: Definition, Types, Scope, & Benefits