Neurophotonics is an interdisciplinary field that involves the study of the brain and nervous system using light-based techniques. It combines principles from neuroscience, physics, optics, and engineering to develop tools and methods for visualizing, manipulating, and understanding the structure and function of neurons and neural circuits.
Technologies in neurophotonics often utilize light to either stimulate or record neural activity with high precision and resolution. This includes techniques like optogenetics, which involves using light to control the activity of specific neurons, and various imaging methods such as functional near-infrared spectroscopy (fNIRS) or two-photon microscopy, enabling researchers to observe and study neural activity in real-time.
Overall, neurophotonics plays a crucial role in advancing our understanding of the brain and holds promise for applications in neuroscience, neurology, and the development of treatments for neurological disorders.
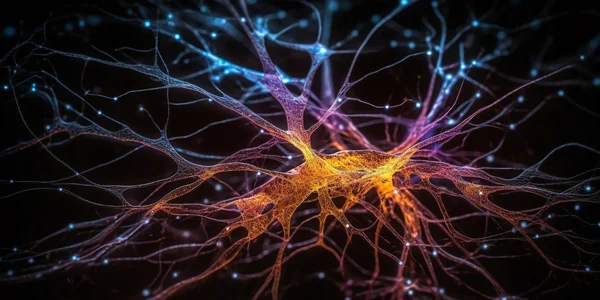
History of Neurophotonics
The history of neurophotonics is intertwined with advancements in both neuroscience and photonics technologies. While the precise term “neurophotonics” might be relatively recent, the foundational concepts and techniques have roots in several developments over the last century or so:
- Early Neural Imaging: The use of light-based methods to visualize neural structures dates back to the development of the microscope. Techniques like staining neurons to enhance contrast and using light microscopy allowed early neuroscientists to observe neuronal structures.
- Fluorescence Microscopy: The advent of fluorescence microscopy in the mid-20th century enabled researchers to visualize specific structures within cells and tissues. This eventually led to the development of fluorescent tags and markers that could label specific neurons or proteins within neurons.
- Optogenetics: One of the pioneering breakthroughs in neurophotonics came in the early 2000s with the development of optogenetics. This technique, which involves using light-sensitive proteins (such as microbial opsins) inserted into neurons, allows researchers to control the activity of specific neurons with light. The work by researchers like Karl Deisseroth, Ed Boyden, and others has been instrumental in this field’s growth.
- Advancements in Imaging Techniques: Techniques such as two-photon microscopy, which enables imaging deep within tissues with high resolution, have been pivotal in visualizing neural circuits and activity in living organisms.
- Functional Imaging Modalities: Functional imaging methods like functional near-infrared spectroscopy (fNIRS) have gained traction. They measure changes in blood oxygenation in the brain to infer neural activity, offering a non-invasive means of studying brain function.
- Development of Light-Based Tools: Continued advancements in photonics technologies, including the miniaturization of devices, the development of advanced light sources, and improvements in imaging and manipulation techniques, have significantly contributed to the expansion and capabilities of neurophotonics.
Over time, the convergence of these technologies and methodologies has given rise to the field of neurophotonics, which focuses on using light-based tools and techniques to understand the structure, function, and dynamics of the brain and nervous system in unprecedented detail. This field continues to evolve rapidly, with ongoing research and innovations shaping its future.
Types of Neurophotonics
Neurophotonics encompasses various techniques and methodologies that utilize light-based technologies to study the brain and nervous system.
Some of the key types or categories of neurophotonics techniques include:
- Optogenetics: This technique involves using light-sensitive proteins (e.g., channelrhodopsins, halorhodopsins) genetically inserted into neurons. By delivering light to these neurons, researchers can selectively activate or inhibit their activity, allowing precise control over neural circuits.
- Multiphoton Microscopy: Techniques like two-photon microscopy enable imaging deep within living tissues with high resolution. This method is particularly useful for studying neural structures and activity in intact brains or live animals.
- Functional Near-Infrared Spectroscopy (fNIRS): fNIRS measures changes in blood oxygenation in the brain, providing indirect but valuable information about neural activity. It’s non-invasive and can be used for brain imaging in both clinical and research settings.
- Optical Coherence Tomography (OCT): Originally developed for ophthalmology, OCT has been adapted for neuroscience. It offers high-resolution imaging of neural tissues, allowing for non-invasive visualization of brain structures.
- Light-sheet Microscopy: This technique allows for rapid imaging of large biological samples with minimal photodamage. It’s particularly useful for imaging neural development in transparent organisms or cleared tissues.
- Photoacoustic Imaging: Combining light and sound, this method can visualize neural structures and activity by measuring the acoustic waves generated when tissue absorbs laser pulses.
- Fluorescence Imaging and Calcium Imaging: Fluorescent dyes and calcium indicators are used to visualize neuronal activity in real-time. Calcium imaging, in particular, tracks changes in calcium ion concentrations, providing insights into neuronal firing patterns.
- Photostimulation Techniques: Laser-based techniques that can deliver precise light stimulation to specific regions or individual neurons, influencing neural activity.
Each of these techniques offers unique advantages and capabilities for studying different aspects of the brain and nervous system. Researchers often combine multiple neurophotonics methods to gain comprehensive insights into neural structure, function, connectivity, and activity.
Facts of Neurophotonics
Absolutely, here are some fascinating facts about neurophotonics:
- Revolutionizing Neuroscience: Neurophotonics has revolutionized neuroscience by offering non-invasive, high-resolution tools to study the brain’s intricate workings in real-time.
- Optogenetics’ Precision: Optogenetics allows scientists to precisely control neural activity using light-sensitive proteins, enabling the manipulation of specific neurons with remarkable accuracy.
- In Vivo Imaging: Techniques like two-photon microscopy enable in vivo imaging, allowing researchers to observe neural activity deep within live brain tissue with cellular-level resolution.
- Clinical Applications: Neurophotonics holds promise for clinical applications, including the development of novel therapies for neurological disorders like Parkinson’s, epilepsy, and depression.
- Understanding Brain Connectivity: Through methods like fluorescence imaging, neurophotonics aids in mapping neural connections and understanding the complex network of interactions within the brain.
- Functional Insights: Functional near-infrared spectroscopy (fNIRS) provides insights into brain function by measuring changes in blood oxygen levels, offering a non-invasive means to monitor brain activity.
- Advancements in Imaging Techniques: Ongoing advancements in imaging technologies within neurophotonics continually improve resolution, depth, and speed, enabling more detailed and comprehensive studies of the brain.
- Emerging Therapeutic Avenues: Researchers are exploring how techniques within neurophotonics, such as targeted photostimulation, might be harnessed for therapeutic purposes, potentially aiding in neural rehabilitation and treatment strategies for brain-related conditions.
- Interdisciplinary Collaboration: Neurophotonics thrives on collaboration across various disciplines, including neuroscience, physics, optics, engineering, and computer science, fostering innovation and novel discoveries.
- Potential for Brain-Machine Interfaces: Continued advancements in neurophotonics may contribute to the development of sophisticated brain-machine interfaces, enhancing communication and control between the brain and external devices.
These facts showcase how neurophotonics has become a pivotal field, offering powerful tools and techniques to unravel the mysteries of the brain, leading to both scientific discoveries and potential clinical applications.
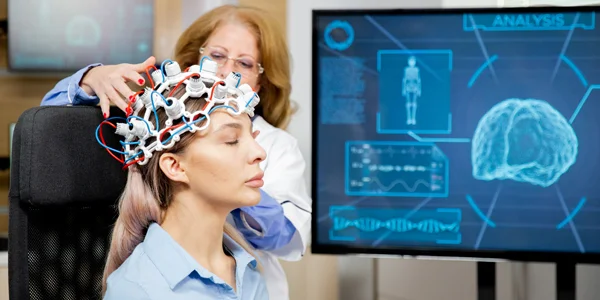
Benefits of Neurophotonics
Neurophotonics offers a wide array of benefits across various aspects of neuroscience research, clinical applications, and technological advancements.
Here are some key benefits:
- High Resolution Imaging: Techniques like two-photon microscopy provide high-resolution imaging, enabling scientists to visualize neural structures and activities with exceptional detail, even deep within living tissues.
- Precise Neural Manipulation: Optogenetics allows for precise manipulation of neural activity using light-sensitive proteins, facilitating targeted control over specific neurons. This has significant implications for understanding neural circuits and behaviors.
- Real-Time Observation: Neurophotonics enables real-time observation of neural activity, allowing researchers to study dynamic processes within the brain, such as synaptic transmission, neural firing patterns, and network connectivity.
- Non-Invasive Monitoring: Methods like functional near-infrared spectroscopy (fNIRS) offer non-invasive means of monitoring brain function in clinical settings, aiding in the diagnosis and assessment of neurological disorders.
- Advancements in Neuroscience: Neurophotonics has significantly advanced our understanding of the brain’s structure, function, and connectivity, contributing to breakthroughs in neuroscience research.
- Potential Therapeutic Applications: Insights from neurophotonics techniques may lead to innovative therapeutic approaches for neurological conditions. For instance, optogenetics-based interventions hold promise for treating conditions like Parkinson’s disease, epilepsy, and depression.
- Interdisciplinary Collaboration: The field encourages collaboration across disciplines, fostering innovation and the development of novel tools and methodologies that benefit both neuroscience and photonics.
- Developmentof Brain-Computer Interfaces: Neurophotonics research contributes to the development of brain-machine interfaces, allowing for better communication between the brain and external devices. This has implications for prosthetics, rehabilitation, and assistive technologies.
- Innovation in Imaging Technologies: Continuous advancements in neurophotonics drive the development of new imaging technologies, improving resolution, sensitivity, and depth of imaging, which, in turn, expands our capabilities in studying the brain.
- Potential for Personalized Medicine: As neurophotonics techniques advance, they may contribute to personalized approaches in diagnosing and treating neurological disorders, tailoring interventions to individual brain characteristics and responses.
Overall, the benefits of neurophotonics span from fundamental discoveries in neuroscience to potential clinical applications, paving the way for innovative solutions in understanding and treating neurological conditions.
What are the challenges in neurophotonics?
Neurophotonics, despite its immense potential, faces several challenges that researchers are actively working to overcome:
- Depth and Penetration: While some techniques offer high resolution, penetrating deep into brain tissue with sufficient clarity remains a challenge. This limits the observation and manipulation of deeper brain regions.
- Invasive Nature of Some Methods: Certain neurophotonics techniques require invasive procedures, such as inserting light-sensitive proteins or probes into neural tissue, which can potentially damage or alter the natural neural environment.
- Specificity and Targeting: Achieving precise targeting of individual neurons or neural circuits can be challenging, impacting the accuracy of stimulation or recording.
- Complexity of Data Analysis: Neurophotonics generates vast amounts of complex data that require sophisticated analysis methods to extract meaningful information. Analyzing and interpreting this data accurately pose significant challenges.
- Biocompatibility and Long-Term Stability: Ensuring that implanted devices or materials used in neurophotonics techniques are biocompatible and stable over extended periods within the brain is critical for long-term studies and potential clinical applications.
- Ethical Considerations: The use of certain techniques, such as optogenetics, raises ethical considerations regarding its application in humans, necessitating careful ethical evaluations and considerations.
- Integration of Technologies: Integrating different neurophotonics technologies to work seamlessly together presents challenges, especially when combining various imaging and manipulation techniques.
- Standardization and Reproducibility: Establishing standardized protocols and ensuring the reproducibility of findings across different labs using neurophotonics methods is essential for advancing the field and building upon previous research.
- Cost and Accessibility: Some neurophotonics tools and equipment can be expensive and require specialized expertise, limiting access for smaller research labs or institutions with fewer resources.
- Translation to Clinical Applications: Bridging the gap between laboratory research and clinical applications remains a challenge, requiring thorough validation, regulatory approval, and adaptation of techniques for safe and effective use in clinical settings.
Despite these challenges, ongoing research efforts are dedicated to addressing these issues, with advancements in technology, methodology, and collaborative initiatives aimed at pushing the boundaries of neurophotonics and overcoming these hurdles.
How does neurophotonics contribute to neuroscience?
Neurophotonics contributes significantly to neuroscience in several ways:
- Visualization of Neural Structures: Techniques like two-photon microscopy offer high-resolution imaging, enabling researchers to visualize and study intricate neural structures with unprecedented detail, aiding in understanding brain anatomy.
- Real-Time Observation of Neural Activity: Neurophotonics allows for real-time observation of neural activity, providing insights into dynamic processes like synaptic transmission, neuronal firing patterns, and network interactions crucial for understanding brain function.
- Mapping Neural Circuits and Connectivity: Methods within neurophotonics facilitate the mapping of neural circuits and connectivity patterns in the brain. This helps in deciphering how different brain regions communicate and work together to process information and generate behaviors.
- Manipulation of Neural Activity: Techniques like optogenetics enable precise manipulation of neural activity, allowing researchers to activate or inhibit specific neurons, which helps in understanding their roles in behavior, cognition, and disease.
- Functional Brain Imaging: Functional imaging methods, including fNIRS, enable non-invasive monitoring of brain function by measuring changes in blood oxygenation, providing valuable information about brain activity in both research and clinical settings.
- Understanding Neural Dynamics: Neurophotonics techniques contribute to understanding the dynamics of neural networks, such as the synchronization of neuronal firing or how changes in neural activity relate to behavior and cognitive processes.
- Insights into Neurological Disorders: Research in neurophotonics contributes to unraveling the mechanisms underlying neurological disorders. By studying neural activity patterns and structural changes, it offers insights into conditions like epilepsy, Alzheimer’s, Parkinson’s, and psychiatric disorders.
- Development of New Technologies: Neurophotonics drives the development of new imaging tools, manipulation techniques, and analysis methods, fostering innovation and expanding the toolkit available for neuroscientists to explore the complexities of the brain.
Overall, neurophotonics plays a pivotal role in advancing our understanding of the brain’s structure, function, and dynamics, contributing to fundamental discoveries in neuroscience and potentially paving the way for new treatments and interventions for neurological conditions.